To feed the world, improve photosynthesis
Inside a balmy greenhouse in central Illinois, a boisterous but focused pair of researchers are seeding experimental plants. The scientists moisten the soil and pack it into pots, then carefully tip tiny dark-brown tobacco seeds out of glass vials. In the months that follow, the researchers will move the plants outside into a field and watch whether they grow bigger or faster than usual—a crucial step toward feeding the world of 2050.
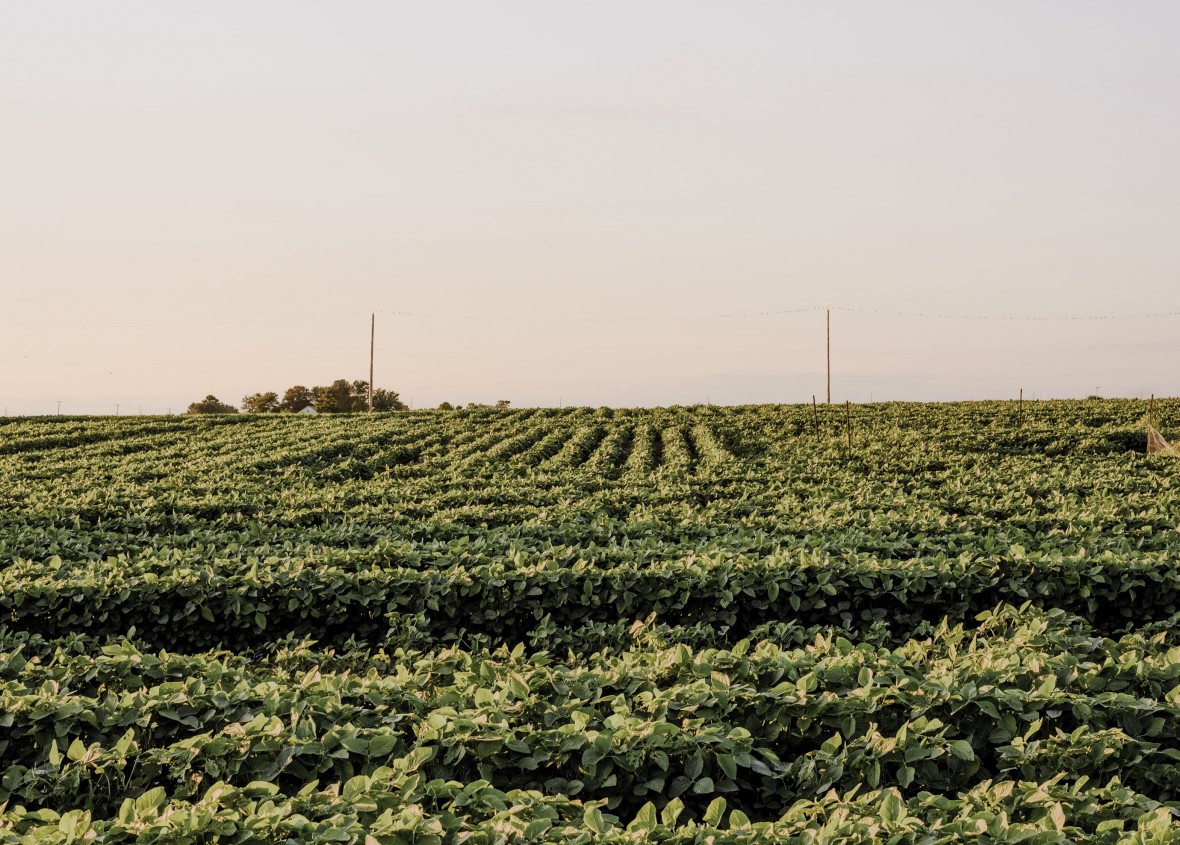
These tobacco plants have been engineered at a more fundamental level than typical biotech crops. The way they conduct photosynthesis has been tinkered with so they convert sunlight and carbon dioxide more efficiently into carbohydrates. If scientists do that in food crops, any given plot of land could produce more food, or produce the same amount of food with less water and fertilizer.
The need is urgent. To feed a growing population, the United Nations projects, worldwide agricultural yields must increase by 50 percent between now and 2050. And that ambitious goal does not factor in the effects of climate change. Plants thrive on carbon dioxide, but very hot days suppress crop yields. In many parts of the world, the rising temperatures and increased droughts caused by climate change will be devastating. And those negative effects “will have the biggest impact on the poor,” says Steve Long, director of the Realizing Increased Photosynthetic Efficiency (RIPE) project, an international consortium based at the University of Illinois at Urbana-Champaign.
The RIPE project, funded by the Bill and Melinda Gates Foundation, is starting with tobacco because it’s relatively easy to genetically engineer. But RIPE’s real aim is to improve the yields of food crops such as cassava and cowpeas, which are important sources of calories and protein in many poor countries. And it is working on much more ambitious changes to plant metabolism than have been made before.
Agronomists have not yet pushed photosynthesis to its limits. That’s in spite of the fact that this 160-step biochemical process is very well studied, and surprisingly inefficient—plants convert less than 5 percent of the energy in sunlight into biomass. A still smaller part of that is invested in the parts of plants people like to eat: seeds, tubers, beans. Modern agriculture has improved yields tremendously thanks to fertilizers, pesticides, and traditional breeding. Now gains are harder to come by. That’s why the RIPE group is targeting inefficiencies in plant metabolism. (Other researchers are trying variations on the same idea; see “10 Breakthrough Technologies 2015: Supercharged Photosynthesis.”)
Last year, RIPE researchers demonstrated for the first time that it was possible to improve crop yields in the field by engineering photosynthesis. By increasing the expression levels of three genes involved in processing light, they improved tobacco yields by 20 percent.
Now the RIPE team is trying to use the same genetic-engineering trick to increase yields in more recalcitrant food crops. Making it happen in cassava falls in part to Amanda De Souza, a postdoc from Brazil.
Genetic engineering of photosynthesis in cassava is a delicate and lengthy process. De Souza opens a petri dish to show off cassava embryos, light-yellow clusters about a millimeter wide. She grows them using tissue plucked from a bud on a full-grown cassava plant. This cluster of cells, called a “callus,” can be infected with bacteria carrying the light-processing genes. Only a few cells will actually take up the genes. Those that do will then be exposed to a hormone cocktail that will drive them to grow a stem and roots.
In cassava, this genetic transformation takes eight to 10 months—that is, if everything goes well. Other key food crops, including rice and cowpeas, are a bit faster.
Down the hall, De Souza opens a closet-like room flooded with artificial sunlight. On shelves, young cassava plants are growing in plastic jars, their roots surrounded by a nutrient gel that will be picked off by hand before the plants can go into the soil.
RIPE’s experimental fields are a 10-minute drive from the labs. In this part of the country, farms mostly grow soybeans and corn. It falls to David Drag, RIPE’s field trials manager, to figure out how central Illinois’s soil can nurture crops like cassava and rice. For one project a collaborator helped him build a rice paddy. But in 2015, he recalls ruefully, he saw one of RIPE’s key projects drown in a severe late-season rainstorm, in spite of the team’s efforts to dig trenches and dams. A year’s work was lost—a humbling reminder that even the most advanced agricultural science is still at the mercy of nature.
These experiments have helped to establish that tobacco plants produce higher yields if they can more quickly turn off production of a “molecular sunscreen” when light levels drop. Plants that perform well in these experiments go on to field trials.
By: Katherine Bourzac || MIT Technology Review
I’m a freelance journalist based in San Francisco, California, and a contributing editor at MIT Technology Review, where I was previously on staff as materials science editor. I write about materials science, computing, and medicine. My favorite nanomaterial is carbon nanotubes and my favorite quasiparticle is the plasmon. I serve on the board of the Northern California chapter of the Society of Professional Journalists. I graduated from MIT’s science writing program in 2004.
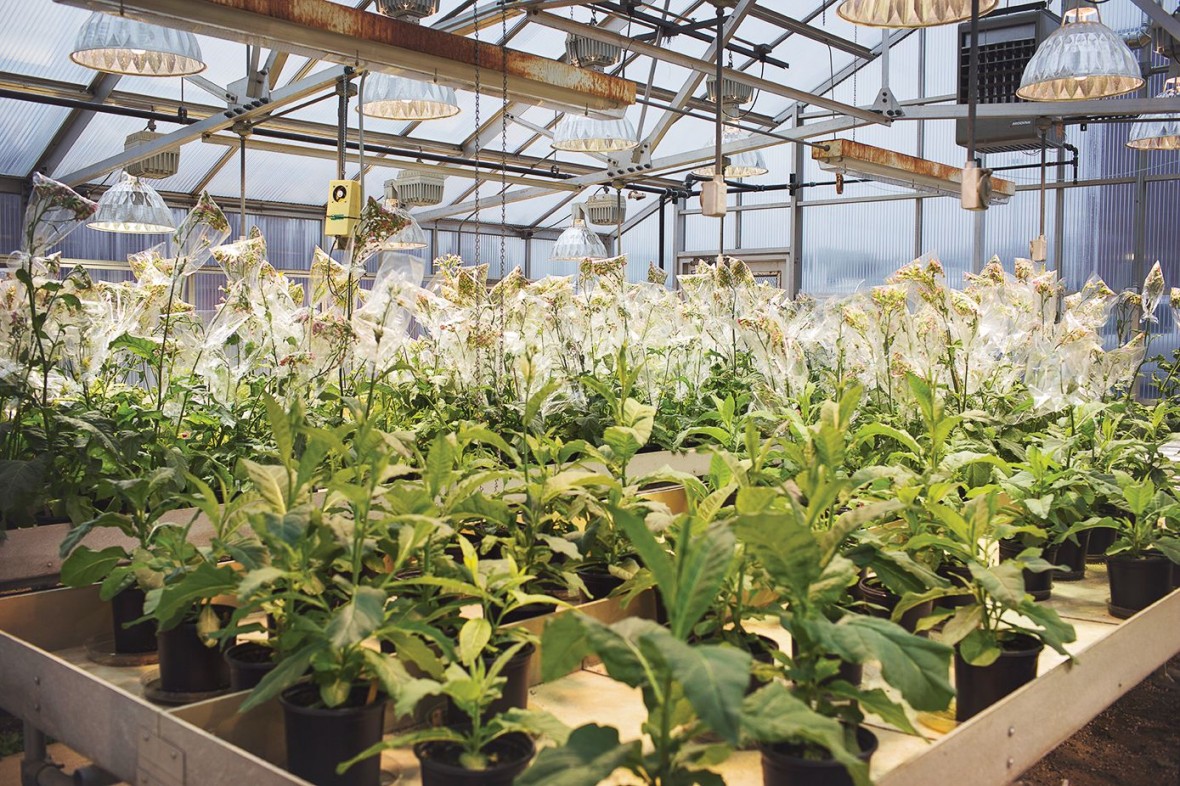
RELATED RIPE OBJECTIVES
Relaxing Photoprotection